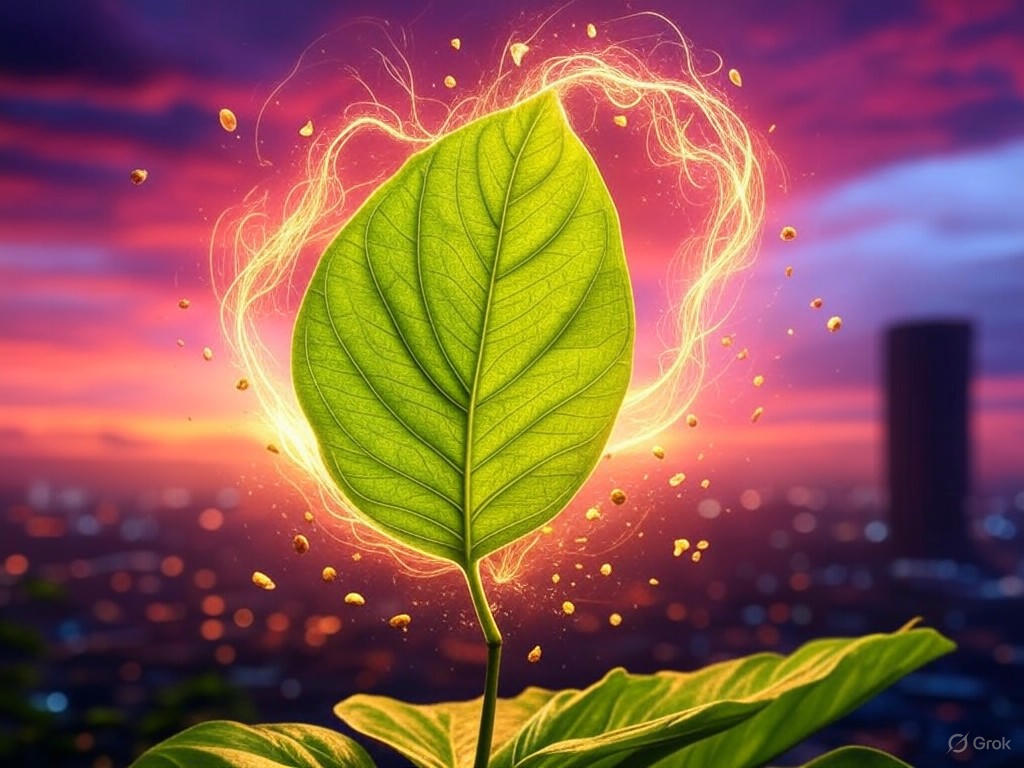
Quantum Effects in Proteins Drive Efficient Energy Transfer
Introduction to Quantum Biology and Energy Transfer
Quantum effects in proteins are at the heart of some of nature’s most efficient processes. Quantum biology, an exciting field, reveals how these subtle quantum phenomena shape vital biological functions like photosynthesis and cellular respiration. Imagine plants turning sunlight into energy with near-perfect precision—it’s all thanks to quantum-driven mechanisms that make biological systems outperform many human-engineered technologies in energy conversion.
Have you ever pondered how a leaf captures light so flawlessly? This efficiency stems from quantum effects in proteins that allow energy to flow with minimal loss, setting the stage for groundbreaking advancements in energy harvesting.
The Nature of Quantum Effects in Proteins
At the molecular level, proteins exhibit remarkable quantum behaviors that enhance energy transfer. Things like quantum tunneling, quantum coherence, and quantum walks enable electrons and protons to navigate barriers that classical physics can’t explain, boosting transfer rates dramatically.
These quantum effects in proteins don’t just happen by chance; they’re finely tuned by evolution to maximize efficiency in everyday biological tasks. For instance, in photosynthesis, energy zips through protein complexes faster than you might think possible, all while keeping losses low.
Quantum Tunneling in Electron Transport Chains
Mitochondria, often called the cell’s power plants, rely on quantum tunneling to produce ATP with incredible efficiency. In their electron transport chains, electrons slip through barriers via quantum tunneling, achieving efficiencies of 60-70%—that’s way better than most engines we’ve built.
Picture this: an electron darting across a membrane as if it were vanishing and reappearing on the other side. This quantum effect in proteins makes cellular respiration so effective, raising the question: could we mimic this in artificial systems to create more sustainable energy sources?
Scientists have observed that this process minimizes energy waste, allowing cells to thrive even in challenging environments. By understanding quantum tunneling, we’re unlocking ways to improve technologies like batteries or fuel cells.
Quantum Coherence and Vibrational Modes
In photosynthetic systems, quantum coherence plays a starring role. This effect lets energy waves interact across protein molecules, spreading excitation energy more effectively and maintaining high transfer efficiency.
Protein vibrations act like subtle guides, helping to preserve this coherence at room temperature. It’s fascinating how quantum effects in proteins turn what could be chaos into a harmonious energy flow, much like an orchestra tuning its instruments.
Ever notice how some plants thrive in dim light? Quantum coherence might be the secret, ensuring energy gets where it’s needed without unnecessary detours.
Quantum Walks and Environment-Assisted Transport
Another layer involves quantum walks, where energy excitations explore protein landscapes in a wave-like manner, potentially speeding up transfer. Environment-assisted transport adds to this by turning surrounding “noise” into a helpful force, guiding energy along the right paths.
These quantum effects in proteins highlight how biology cleverly adapts quantum principles for real-world use. In light-harvesting complexes, this means energy reaches reaction centers quickly and accurately, even amidst molecular bustle.
Is it possible that what we see as random in nature is actually a sophisticated quantum strategy? Researchers think so, and it’s changing how we design materials for solar energy.
Debate Over Quantum Walk Contributions
There’s ongoing discussion about how much quantum walks truly accelerate energy transfer. Some studies suggest classical models, enhanced with quantum elements, can explain the efficiency we observe.
Yet, the prevailing view is that quantum effects in proteins strike a balance between speed and precision. For example, in bacteria like purple sulfur ones, this fine-tuning ensures energy is used wisely rather than wasted.
If you’re curious, think about a busy city where traffic flows smoothly despite obstacles—quantum walks might be nature’s version of smart routing.
Protein-Based Models Mimicking Natural Energy Transfer
Researchers are now creating protein-based models that copy these natural processes. Using modified proteins from things like tobacco mosaic viruses, scientists build structures that guide energy from one point to another, just like in plants.
These models demonstrate how quantum effects in proteins can inspire bio-inspired tech, such as advanced solar panels. Imagine harnessing sunlight as efficiently as a leaf—it’s not science fiction anymore.
One practical tip: if you’re into DIY science, consider exploring simple experiments with light-sensitive materials to see energy transfer in action. This could be a fun way to grasp the basics of quantum effects in proteins.
Implications and Future Directions
The insights from quantum effects in proteins are paving the way for revolutionary energy technologies. By studying quantum coherence and tunneling, we’re developing materials that could make solar energy cheaper and more effective.
This knowledge deepens our appreciation for biological efficiency and opens doors to fields like molecular electronics. For instance, what if we could create devices that mimic mitochondria for portable power?
A hypothetical scenario: In the near future, your phone might charge using quantum-inspired proteins, lasting days on a single exposure to light.
Challenges in Harnessing Quantum Biology
- Quantum coherence often fades quickly at body temperatures, making it tough to apply in real-world devices.
- The complex interactions within proteins demand sophisticated tools for study and replication.
- Blending quantum and classical dynamics is key to optimizing artificial systems, and researchers are actively tackling this.
Despite these hurdles, overcoming them could lead to breakthroughs. Here’s a strategy: Start with simulations to model quantum effects in proteins before building prototypes.
Conclusion
Quantum effects in proteins fundamentally enhance energy transfer, allowing life to function with such grace and efficiency. From tunneling electrons to coherent waves, these mechanisms push beyond classical limits, powering everything from plants to our own cells.
As we continue to unravel these mysteries, the potential for innovation is vast. What are your thoughts on how quantum biology might shape the future—could it help solve our energy crises?
We’d love to hear from you in the comments below. Share this article if it sparked your interest, and explore more on quantum topics through our related posts.
References
1. “Quantum biology” from Wikipedia: https://en.wikipedia.org/wiki/Quantum_biology
2. Article on quantum effects in biological systems: Frontiers in Quantum Science
3. Review on quantum effects in the brain: AIP Publishing
4. Study on quantum coherence in photosynthesis: PubMed
5. ArXiv paper on quantum biology: arXiv
6. ACS publication on energy transfer: ACS Journals
7. Review on quantum effects in chemistry: ACS Chemical Reviews
8. CECAM workshop details: CECAM
quantum effects in proteins, energy transfer efficiency, quantum biology, photosynthesis, quantum coherence, quantum tunneling, biological energy systems, protein dynamics, quantum walks, energy harvesting